Ryan Crislip, PhD
Postdoctoral Fellow
University of Florida
The circadian field has gained recent notoriety from three distinguished scientists (Jeffrey Hall, Michael Rosbasch, and Michael Young) being awarded the Nobel Prize in Physiology or Medicine in 2017 for their isolation and characterization of the circadian gene Period in fruit flies. Amazingly, the circadian clock is found and is similar in many different species from fruit flies to mice and to humans. Within mammals, the circadian clock plays a role in many bodily functions, so it is no surprise that dysfunction of the clock is associated with many biological disorders such as hypertension and other cardiovascular complications. Understanding circadian clock function will provide critical information for overall human physiology and pathophysiology.
Most physiological processes in the human body exhibit 24-hour rhythms. The suprachiasmatic nucleus within the brain serves as the central circadian clock and plays a major role in conducting these rhythmic functions. The central clock uses light to entrain an intricate transcription translation oscillating loop, which in turn sends signals throughout the body. These circadian oscillations are generated by a set of core circadian proteins that are transcription factors:
- Core proteins circadian locomotor output cycles kaput (CLOCK)
- Brain and muscle ARNT-like 1 (BMAL1)
- Period (Per)
- Cryptochrome (Cry)
Individual cells throughout the body also display these oscillating loops that work in harmony within each organ, creating peripheral tissue clocks. One example is how liver function relies heavily on the circadian oscillations exhibited by hepatic cells. Dysfunction in these circadian clocks have been linked to several disorders, such as the acceleration of fatty liver disease and hepatitis. The importance of the circadian clock is also observed in other peripheral organs. Here, we examine the role of major components of the circadian clock within cells of the kidney that are responsible for solute handling.
The formation of urine is achieved by glomerular filtration, tubular reabsorption and secretion, and excretion. The composition of excreted urine is associated with the body’s homeostasis of solutes and acid/base balance. Kidney function, and many hormonal systems that influence function, exhibit daily rhythms (Figure 1). Several studies have examined how clock proteins within the kidney contribute to blood pressure control and urine formation. The use of genetically engineered rodents, which lack certain core circadian proteins, has greatly advanced the understanding of the relationship between clock proteins within cells of the kidney and solute handling.
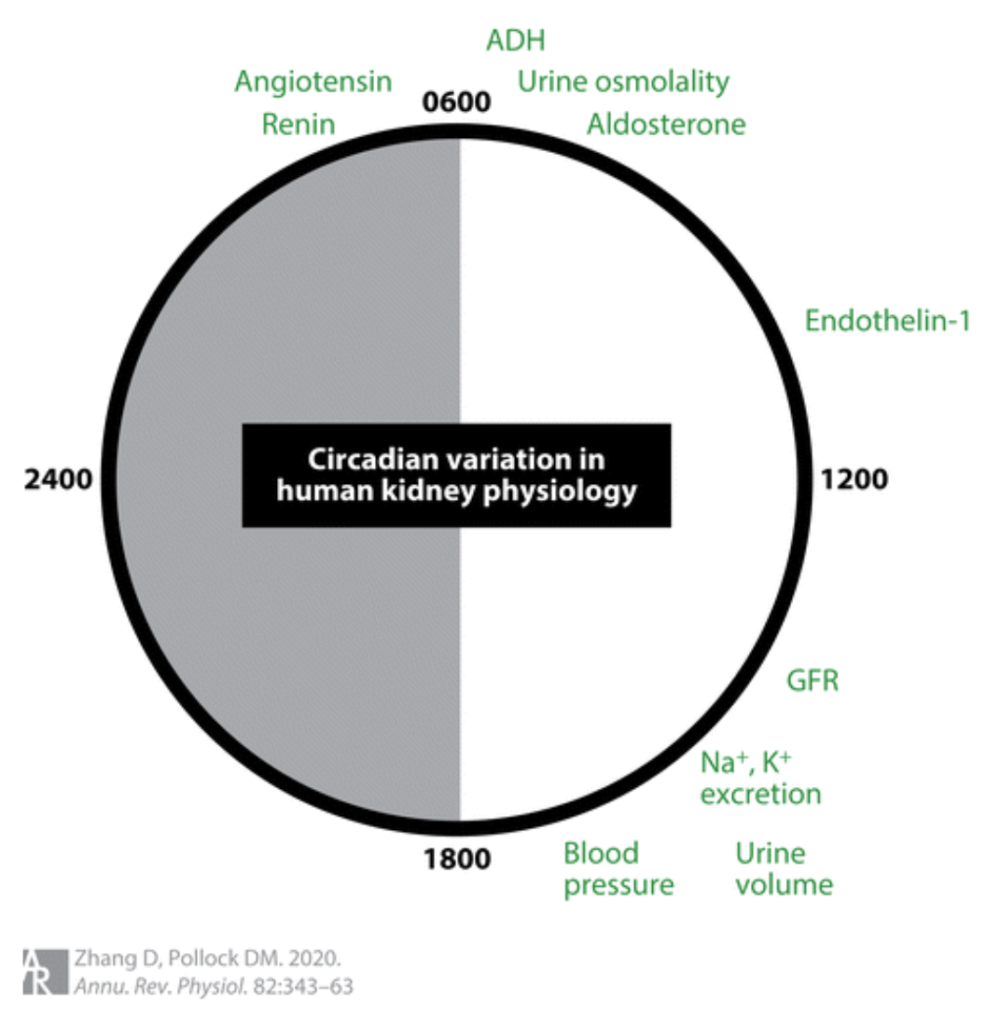
Blood pressure also exhibits a circadian rhythm with higher pressures during the active period and a ‘dip’ in pressure during the inactive period. Normally this dip during the inactive period is 10-20% lower than the active period. Disruptions in the mechanisms that control blood pressure can lead to abnormalities in rhythms. Individuals that do not exhibit the drop in blood pressure during the inactive period are non-dippers. Non-rising phenotypes are seen when the blood pressure does not increase during the active period.
One of the most studied circadian factors within the kidney is BMAL1, and its role in electrolyte balance and blood pressure regulation. Completely knocking out BMAL1 in mice lowers blood pressure and leads to a non-rising blood pressure phenotype. Additional studies have used kidney-specific knockout (KO) mouse models and looked at its effects on blood pressure and electrolyte handling. Loss of BMAL1 in renin-lineage cells, the whole nephron epithelium, or the distal nephron leads to lower blood pressure. Additionally, it was observed that animals with renin-lineage cells that lack BMAL1 have a higher glomerular filtration rate (GFR) and excrete more sodium in their active period than control mice. Similarly, we have shown that distal-nephron-specific KO of BMAL1 leads to greater sodium excretion after a low potassium dietary challenge (0.25% NaCl and 0.0001% K+). This effect was only seen in male mice, however, as there was no effect in both blood pressure and solute handling in female distal-nephron-specific KO mice. Overall, these studies demonstrate that renal BMAL1 contributes to sodium balance and blood pressure control. Additionally, there is a sex-specific response to the actions of BMAL1 where females are protected from the changes reported when BMAL1 is absent in males. Dietary treatments that promote sodium retention may lead to increased understanding of mechanisms involved.
Other core clock components have also been linked to the regulation of blood pressure and electrolyte balance. Although these studies use global KO models, changes in kidney sodium transporter expression have also been reported. As seen with BMAL1 KO models, other components of the clock seem to be important in the blood pressure response to various diet manipulations. Our group has shown that male global Per1 KO mice had higher blood pressure (~10 mmHg) during their active period and developed non-dipping hypertension following high salt (4% NaCl) plus mineralocorticoid treatment (75 µg/g). Female Per1 KO mice, on the other hand, maintained normal rhythm and had no change in blood pressure magnitude. Per1 has been shown to regulate the expression of sodium transporters in the kidney collecting duct, which may contribute to the observed response between male and female Per1 KO mice. Additional studies have examined a Per1 homolog, Per2. Interestingly, mice lacking Per2 have lower blood pressure during their active phase. The circadian rhythm of blood pressure is lost in Per2 KO mice (also in Per1, 2, and 3 triple KO mice) following a low salt diet treatment (0.01-0.02% NaCl). Clock mutant mice have demonstrated blunted night/day differences in blood pressure. Furthermore, Clock KO mice display increased sodium excretion. Although global Cry1/2 KO mice had no change in blood pressure averages, they did lose circadian rhythm in blood pressure. However, following a high salt diet treatment (3.15% Na+), Cry KO mice demonstrated higher blood pressure than treated controls. All core clock KO mouse models demonstrate a blood pressure phenotype. There is also evidence of a sex difference in the blood pressure response in some of these KO models, however, most studies use only males and do not include female data. Identifying the mechanisms in play on how the circadian clock contributes to electrolyte handling and blood pressure control may lead to potential targets for treatment in patients with disrupted circadian clocks.
Animal Model | Blood/Mean Arterial Pressure Changes | Electrolyte Balance Changes |
Global BMAL1 KO | ⬇ MAP Loss of BP dip during inactive period | N/A |
Renin lineage BMAL1 KO | ⬇ BP | ⬆ Active period Na+ excretion ⬇ Inactive period Na+ excretion |
Nephron BMAL1 KO | ⬇ Systolic BP | N/A |
Distal tubular and collecting duct BMAL1 KO | ⬇ BP only in males | ⬆ Na+ excretion with low K+ diet only in males |
Smooth muscle Bmal1 KO | ⬇ BPLoss of BP dip during inactive period | N/A |
Global Per1 KO | Non-dipping hypertension (high Na+ diet w/ DOCP) | N/A |
Female Global Per1 KO | Protection from non-dipping hypertension (high Na+ diet w/ DOCP) | N/A |
Global Per2 Mutant | ⬇ Diastolic BP | N/A |
Global Per2 KO | Non-dipping hypertension (low Na+ diet) | N/A |
Global Clock Mutant | ⬆ BP during inactive period⬇ BP dip | N/A |
Global Clock KO | ⬇ BP | N/A |
Global Cry1/Cry2 KO | Salt-sensitive hypertension | N/A |
Circadian regulation of daily rhythms in blood pressure regulation and solute handling are well established, however, the contribution of the renal peripheral clock to these physiological processes needs further investigation. Many kidney functions including GFR, electrolyte excretion, and renal blood flow all exhibit a daily rhythm, so it is important to consider this variation when performing tests or interpreting results in a clinical setting. Additionally, some patients with chronic kidney disease exhibit non-dipping blood pressure and disrupted circadian rhythms in urine output. Shift work and individuals who are exposed to light at night are at risk of disrupting their circadian rhythms. Therefore, we need to increase our understanding of the clock at a molecular level and its effects on pathophysiology, particularly within the kidney.
Reviewed by: Elinor Mannon, Kelly Hyndman, PhD, Matthew Sparks, MD, FASN, FAHA
If you enjoyed reading this post and would like to learn more about the circadian clock in the kidney, consider checking out this post on RFN: